Table of Contents
By: Sarah Choe, Tianjia Cui, Elias Espinosa, Rachel Guan, Tim Wang
Comparison of Immune Response for SARS-CoV-2 Naive and Experienced Individuals Following Two Doses of BNT162b2
Introduction
It is without doubt that vaccines save lives. Pfizer-BioNTech developed the BNT162b2 vaccine against COVID-19, with the goal of eradicating SARS-CoV-2, and to reduce transmission rates worldwide (Pfizer, n.d.). As of March 2, 2022 there have been 437,333,859 confirmed COVID-19 cases, with 10,585,766,316 vaccines administered globally (WHO, 2022). Perhaps, there is a difference in immune response after the first and second dose of BNT162b2 for SARS-CoV-2 experienced and SARS-CoV-2 naive individuals due to post-infection immunity (Samanovic et al., 2021).
Post-Infection Immunity
Post-infection immunity, also known as natural immunity, provides an explanation for the observed immunological protection that is present to prevent re-infection for individuals who have previously been faced with a specific antigen (Spicer et al., 2022). Spicer et al. (2022) suggest that 80.3% of SARS-CoV-2-experienced individuals were protected against subsequent re-infections of SARS-CoV-2 due to post-infection immunity. After an individual is infected by SARS-CoV-2, B cells differentiate into long-living plasma cells to produce anti-SARS-CoV-2 antibodies against SARS-CoV-2 (Turner et al., 2021a). It was found that after 7 months of infection, anti-SARS-CoV-2 antibodies were gradually increasing, and remained detectable after 11 months (Turner et al., 2021a). Turner et al. (2021a) also discovered that memory B cell levels were elevated as a result of SARS-CoV-2 infection.
Immunological Memory of Vaccines and Post-Infection Immunity
The BNT162b2 is a mRNA based vaccine that encodes the spike protein of SARS-CoV-2, the virus responsible for COVID-19 (CDC, 2022). From here, patients are exposed to the SARS-CoV-2 antigen, thus, initiating an immune cascade to induce immunological memory (CDC, 2022; Samanovic et al., 2021). In a similar manner, SARS-CoV-2 experienced individuals were also exposed to the antigen, thus causing a similar immunological cascade to induce immunological memory (Turner et al., 2021a).
Objective
The paper “Robust immune responses after one dose of BNT162b2 mRNA vaccine dose in SARS-CoV-2 experienced individuals” by Samanovic et al. (2021) aims to compare the difference in immune response for SARS-CoV-2 experienced and SARS-CoV-2 naive individuals following the first and second dose of BNT162b2.
Material and Methods
Study Design
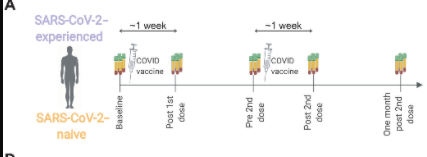
Figure 1: Diagram of study design, whereby vaccine injection and blood serum collections can be visualized (Samanovic et al., 2021).
To test the effect of prior infection and vaccination on immunologic response, Samanovic et al., 2021 conducted a study design that would allow for proper analysis. The researchers recruited 36 individuals and separated them into two groups; COVID experienced and COVID naive. 15 participants were allocated into the experienced group and 21 into the naive group. The ages of participants ranged from 21-to 65. For both cohorts, participants were given two doses of the BNT162b2 mRNA vaccine and peripheral immune responses were measured and analyzed. Blood samples were collected periodically throughout the study, starting at the baseline, just days before the 1st dose. Then, Post 1st dose (6 to 13 days after vaccination), Pre 2nd dose (immediately before second vaccination), Post 2nd dose (7 to 12 days after second vaccination), and One-month post 2nd dose (about 4 weeks after second vaccination). Samanovic et al., 2021 chose to collect samples 1 week after the second vaccination because that was the peak of the humoral response after mRNA vaccination. Using the cultivated red blood cells in conjunction with clinical assays, the immunogenic responses of both cohorts can be adequately studied and interpreted.
Flow Cytometry
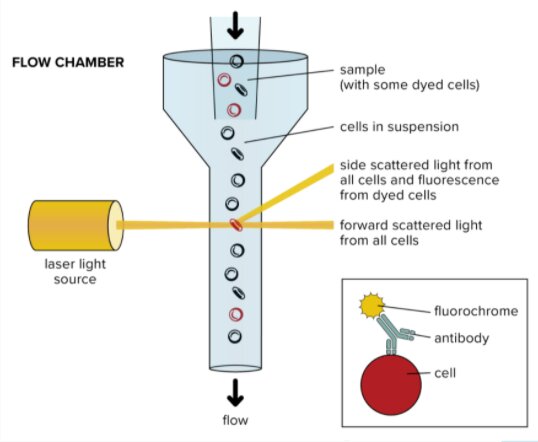
Figure 2: A diagram depticting all the step in flow cytometry (Nanocellect, 2021).
Samanovic et al., 2021, first wanted to determine the phenotype of circulating T cells that were activated upon vaccination. They performed flow cytometry to do so. Flow cytometry is a technique used to detect and measure the characteristics of a population of cells of particles. An aliquot of cells is suspended in a fluid and is then passed through a narrow channel. The cells descend the column single file at very high speeds. As they descend, a laser shines onto the cells, producing unique and varying scattering patterns behind them. These initial events are characteristic of the size and shape of the cell. These patterns reflect cell types and can be analyzed and quantified in computer software programs. Digital read-outs can be displayed on a computer screen, allowing researchers a greater understanding of T cell phenotypes (nanocellet, 2021).
Activated Induced Markers (AIM)
Antigen-specific circulating CD4+ T follicular helper cells are critical for the development of antibody responses. Thus detection of antigen-specific Tfh cells is important for both understanding vaccine efficacy and tracking disease progression (Reiss et al., 2017). Samanovic et al., 2021 then wanted to find means of analyzing antigen-specific cells which did not secrete cytokines, as they are hard to find conventionally. To do this, they used a relatively new, and highly specific assay called Activated Induced Marker (AIM) assay. This assay utilizes the upregulation of TCR stimulation-induced surface markers to find expression rates, a number of cells of specific cytokine, and non-cytokine-producing T cells. To perform an AIM assay, a sample of blood must be suspended in a peptide pool which serves to stimulate viral entry conditions. Then the activation markers can be stained in different colors using a fluorochrome. Finally, the luminescent T- cells can be placed into a flow cytometry device for data analysis. Research done by Kovarik et al., 2021 demonstrates how effective AIM analysis is at characterizing and quantifying antigen-specific immune cells for research purposes. They use AIM assay to detect the early onset of COVID-19 infection.
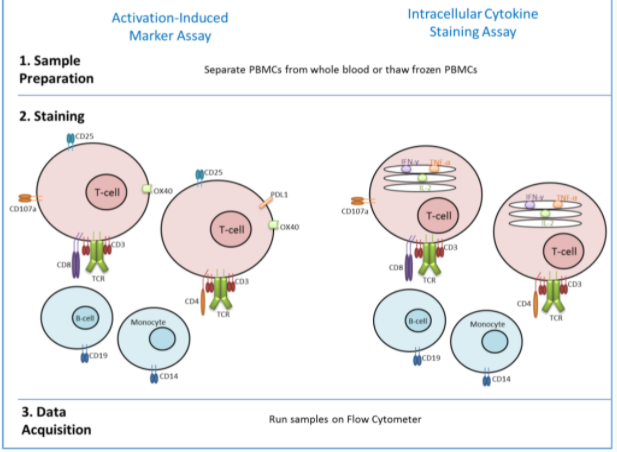
Figure 3: Activated Induced Markers assay, diagram depicting how it works. (Flaxman, A., & Ewer, K. 2018)
ELISpot
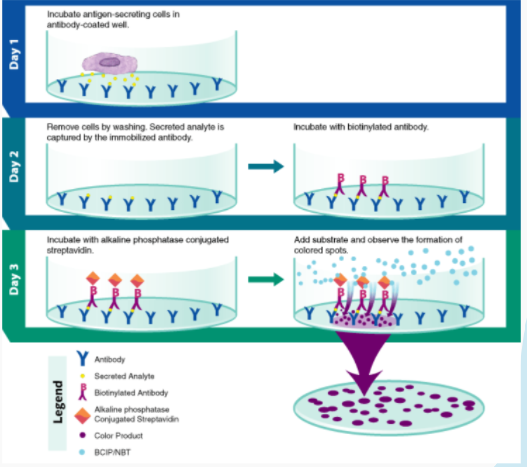
Figure 4: A diagram depticting all the stepw in flow cytometry (ELISpot Assay, 2022).
The ELISpot assay, also known as enzyme-linked immunosorbent assay, measures the amount of cytokine-secreting cells within the blood at a single cell level while also quantifying the number of antigen-specific B cells that have recently been activated (ELISpot assay, 2022). Samanovic et al., 2021 use the ELISpot assay to tackle the research question “Are antigen-specific B cells induced by vaccination influenced by prior exposure to the virus?” To perform the ELISpot assay an antibody specific for the chosen analyte is coated onto a well/microplate. The collected peripheral red blood cells are pipetted into the microplate and are placed in a 37 ° incubator overnight. The stationary immobilized antibodies, in the immediate vicinity of the secreting cells, bind the analyte. The microplate is then rinsed to get rid of all unbound substances. Then a biotinylated polyclonal antibody specific to the analyte is added and binds to the antibody-analyte complex. Another wash is conducted to rinse away any unbound material and alkaline-phosphatase conjugated to streptavidin is pipetted into the microplate. Again, the complex is rinsed. The substrate solution AEC is lastly added to the mixture and a blue-black composition is formed on the surface of the plate.
ELISA
Antibody avidity is described as the strength with which an antibody binds to a complex antigen. Samanovic et al., 2021 decided to test anti-S1 IgG antibodies using an enzyme-linked immunosorbent assay, known as ELISA. In (1)’s paper, they use a direct ELISA which can be described as follows. Ninety-six well plates were coated with S1, spike protein. Human IgA, IgM, and IgG are conjugated with horseradish peroxidase, a staining agent (Rudnick & Adams, 2009). This mixture binds to the spike proteins and a deep color is formed. This can be detected and reprised as data analyzed by a computer.
.
Results
Robust T cell responses are observed after mRNA vaccination
The first section of the article’s results explores T cell response after vaccination. In response to vaccination, the researchers first wanted to identify the phenotypes of the circulating T cells. This was determined for all participants by using high-dimensional spectral flow cytometry longitudinally. The researchers then performed cluster analysis and t-distributed stochastic neighbor embedding representation of all non-naive CD8+ T cells collected from all time points of all participants. After 29 clusters were identified, only cluster 12 increased in abundance at the Post 2nd dose time point in comparison to Pre 2nd dose. This was based on the data from prior studies that T cell responses would be most present after the second dose (humoral response also peaked 1 week after second vaccination). They determined that the many proteins expressed from the cells in cluster 12 were associated with activation, such as Ki67, CD38, and inducible costimulator ICOS (Samanovic et al., 2021). These cells were then longitudinally assessed by manual gating for Ki67 and CD38, revealing that there was robust induction of Ki67+CD38+ CD8+ T cells one week after each vaccination. In comparison to baseline Ki67+CD38+ CD8+ T cell frequencies, there was a median 1.9-fold increase in induction for SARS-CoV-2 naive and SARS-CoV-2 experienced individuals, one week after the first dose respectively. In contrast, the second vaccine dose induced a 2.2-fold and 3.3-fold increase in SARS-CoV-2–naive and SARS-CoV-2–experienced individuals relative to the pre 2nd dose time point. Additionally, analysis of time as a continuous variable produced an inconsistent pattern, removing the possibility for differential timing of the T cell responses between the two groups. Furthermore, Ki67+CD38+ CD8+ T cells were confirmed to express granzyme B, suggesting the potential for strong cytotoxicity, while repeat exposure to SARS-CoV-2 antigens induced responses with memory kinetics. For these specific findings, the data indicates that cytotoxic CD8+T cell responses are associated with mRNA vaccination in both cohorts (Samanovic et al., 2021).
After evidence of the association of cytotoxic CD8 T cell response with mRNA vaccination in both cohorts, circulating CD4+ T cells were then investigated for similar effects. Cluster analysis of non-naive CD4+ T cells produced 22 clusters, of which only clusters 13 and 21 increased in abundance after a second dose. Moreover, high expression of Ki67, CD38, and ICOS was associated with cluster 13. Once again, longitudinal analysis indicated a 1.9-fold increase of the induction of Ki67+CD38+ CD4+ T cells in SARS-CoV-2–naive adults compared to baseline, after the first vaccination. Post 2nd dose, there was a 1.3-fold increase relative to the Pre 2nd dose time point. However, this was contrasted by muted CD4+ responses observed in SARS-CoV-2–experienced adults. Once again, The possibility of differential timing of CD4+ T cell responses between the two cohorts was also disregarded due to inconsistent patterns. Furthermore, the two participants with recent COVID-19 infection displayed the strongest CD8+ T cell dynamic responses after each dose, while their CD4+ T cell response remained muted (Samanovic et al., 2021).
With the results suggesting an induction of activated CD4+ T cells after vaccination in SARS-CoV-2 naive adults only, the researchers then investigated the correlation between activated CD4+ and CD8+ T cell responses. It was determined that activated CD4+ and CD8+ responses after the first dose of vaccine had a strong positive correlation, whereas a weak correlation was observed after the second vaccination in SARS-CoV-2–naive adults. This was contrasted by modest correlation and no correlation after the first and second vaccine dose respectively, for SARS-CoV-2–experienced adults. Demographic variables such as age were also considered in the analysis due to previous studies associating reduced vaccine immunogenicity with increasing age. Accordingly, no correlation was observed between age and activated CD8+ T cell responses, however, negative correlations were observed for activated CD4+ T cell responses with participant age after both vaccine doses. As a result, the potential for reduced CD4+ T cell response with aging after vaccination, highlights the need for further understanding of the effect of aging on mRNA vaccine-induced immune response(Samanovic et al., 2021).
Figure 6: Labelled G on the left: Example flow cytometry plots showing CD4+ T cell expression of Ki67 and CD38 in a SARS-CoV-2–experienced participant. Red numbers indicate frequency. Labelled H on the right: Summary data for Ki67+CD38+ expression in CD4+ T cells shown by cohort which comprise of SARS-CoV-2–naive (orange, n = 19) and SARS-CoV-2–experienced (purple, n = 14) participants. Thick lines represent median of values.
Antigen-specific T cell responses are induced by mRNA vaccination
On the basis that T cell responses were observed based on phenotypic changes in both groups, the researchers could hypothesize that the vaccination induces antigen-specific T cell responses. With prior studies showcasing T cells that produced TNF- or IFN-γ after mRNA immunization. This prior study assessed the induction of TH1 T cell responses from the BNT162b1 vaccine and reported the activation of virus-specific CD8+ and CD4+ T cells and the robust release of the cytokine IFNy (Sahin et al., 2020). As a result, the study first investigated cytokine production after vaccination. This was achieved by overnight stimulation of peripheral blood mononuclear cells (PBMCs) with SARS-CoV-2 spike protein peptide pools across all time points. Intracellular staining was then used for IFN-γ or TNF. At baseline, Anti-spike protein T cell responses were observed and consistent with reports of pre-existing cross-reactivity. After vaccination, progressively higher frequencies of CD8+ and CD4+ T cells producing cytokines were observed in response to peptide stimulation among SARS-CoV-2–naive individuals. In contrast, only stable frequencies of CD8+ and CD4+ T cells producing the cytokines that were observed among SARS-CoV-2–experienced adults. These results allowed the study to conclude that antigen-specific T cells were at least induced by vaccination for SARS-CoV-2–naive adults. The study then moved onto utilizing other methods for detecting antigen-specific cells. In this case, activation-induced markers (AIM) were used to study antigen-specific T cells that may not produce cytokines. After overnight stimulation of PBMCs with peptide pools for the SARS-CoV-2 spike protein, the induction of CD137+IFN-γ+ CD8+ after vaccination in SARS-CoV-2– naive adults were detected, but not in SARS-CoV-2–experienced adults. The induction of CD4+ T cells by vaccination was also examined and those co-expressing CD69 and CD200 increased ninefold at one month post second vaccination compared to baseline for SARS-CoV-2–naive adults. For SARS-CoV-2–experienced adults, there was no increase. The co-expression of additional surface proteins such as OX40 and CD137 were also assessed and produced similar results. These results allow the study to conclude that spike protein-specific T cell responses were induced in SARS-CoV-2-naive adults, but did not increase in SARS-CoV-2–experienced adults after vaccination (Samanovic et al., 2021).
Figure 7: A flow cytometry plot shown at all time points for CD8+T cells isolated from SARS-CoV-2–naive and SARS-CoV-2–experienced individuals evaluated for the expression of IFN-γ and CD137 (labelled A). Accompanying the plot is a summary graph of the flow cytometry data(labelled B). IFN-γ and CD137 expression are shown for SARS-CoV-2–naive (orange, n = 6) and SARS-CoV-2–experienced (purple, n = 6) participants. Thick lines represent median of values
Differential induction of circulating T follicular helper cells after vaccination was observed between those with and without prior history of COVID-19
Protection by vaccination is often conferred by the induction of class-switched, affinity-matured antibody response. Based on the differences of CD4+ T cell responses between both cohorts after vaccination, CD4+ T cell responses relevant to antibody response were studied next (Samanovic et al., 2021). CD4+ T follicular helper cells serve to aid the maturation of B cells within germinal centers. However, due to the location of spike protein-specific germinal center B cells aspirating to the axillary lymph after mRNA vaccination, it is required to study lymphoid tissue which is difficult to perform routinely. As a result, the study has decided to focus on a circulating Tfh-like (cTfh) subset with similar phenotypic, transcriptional, epigenetic, and functional characteristics to lymphoid Tfh. Previously, the same researchers had determined that vaccination induced antigen-specific ICOS+CD38+ cTfh which exhibited memory kinetics. Further support can be gained from the mRNA influenza vaccination in nonhuman primates which also demonstrated similar activated cTfh responses in other studies (Samanovic et al., 2021). One study reports the development of protective antibody responses through B cells correlating with emerging blood ICOS+CXCR3+CXCR5+CD4+ T cells upon seasonal vaccination (Bentebibel et al., 2013). Meanwhile, another study highlights correlation between cTfh changes related to age with reduced influenza response (Herati et al., 2014). With the supporting data from these previous studies, the researchers decided to evaluate activated cTfh in humans after SARS-CoV-2 mRNA vaccination, which had not been investigated yet (Samanovic et al., 2021).
Evidence of cTfh responses were examined at all time points. For SARS-CoV-2–naive-adults, ICOS+CD38+ cTfh cells increased after vaccination and peaked one week after the second vaccination. However, a similar induction was not seen in SARS-CoV-2–experienced adults at either vaccination. Next, prior studies demonstrated expression of CXCR3 by antigen-specific ICOS+CD38+ cTfh after influenza vaccination (Samanovic et al., 2021). Accordingly, the subset of ICOS+CD38+ cTfh that expressed CXCR3 were investigated. The findings demonstrated a 2.1-fold induction of CXCR3+ cells among ICOS+CD38+ cTfh cells for SARS-CoV-2–experienced adults after the first vaccination and a 2.0-fold increase among SARS-CoV-2–naive adults likewise. However, in both cohorts, the change in CXCR3 expression in ICOS+CD38+ cTfh after one week of the second vaccination was minimal. Meanwhile, SARS-CoV-2–experienced participants with recent infection exhibited a dynamic induction of XCR3+ cells among ICOS+CD38+ cTfh cells after each vaccine dose (Samanovic et al., 2021). In addition to what was previously done, the AIM assay was utilized to also assess antigen-specific cTfh induced by vaccination. For the AIM assay, Spike protein–specific activated cTfh were identified as CD4+ T cells expressing CXCR5 and programmed cell death protein 1 (PD-1), with high expression of CD38 and coexpression of CD69 and CD200 after overnight stimulation with a spike peptide pool. For this subset, a 14.5-fold induction of cells was observed in SARS-CoV-2–naive adults at the Post 2nd dose time point relative to baseline. Similar to the trend with CXCR3+ICOS+CD38+ cTfh, no increase was determined for SARS-CoV-2–experienced adults at the same time interval. Together, the results indicate the induction of spike protein-specific cTfh in naive adults after mRNA vaccination (Samanovic et al., 2021).
Figure 8:Labelled E on the left: PBMCs were stimulated with overlapping SARS-CoV-2 spike peptides for 20 hours followed by phenotypic analysis. Assessment of CD38hi cTfh for expression of CD69 and CD200. Red numbers indicate frequency. Labelled F on the right: Summary plots for CD69 and CD200 coexpression among CD38hi cTfh for both cohorts, represented as SARS-CoV-2–naive (orange, n = 6) and SARS-CoV-2–experienced (purple, n = 6) participants. Thick lines represent median of values.
The researchers then investigated if the cTfh responses correlated with the Ki67+CD38+ CD4+ response. For SARS-CoV-2–naive adults, their ICOS+CD38+ cTfh had positive correlations with Ki67+CD38+ CD4+ T cells for the fold change at Post 1st dose compared to baseline and at Post 2nd dose relative to Pre 2nd dose. Contrary to SARS-CoV-2–experienced adults, they had correlated positively after the first dose but showed no correlation after the second dose. Furthermore, there was a negative correlation with age for both cohorts as observed for activated CD4+ responses (Samanovic et al., 2021). Additionally, plasmablasts, CD21lo B cells, and CD71+ B cells among the other well-established cellular correlates of the humoral response were examined, only to show little or no induction of these subsets in both cohorts longitudinally. Lastly, the reported plasma biomarker of early germinal center activity, plasma CXCL13, also did not change after vaccination in either cohort (Samanovic et al., 2021).
In summary, these findings indicated the antigen-specific induction of ICOS+CD38+ cTfh after vaccination with notable differences between cohorts. While the frequency of ICOS+CD38+ cTfh increased continually for SARS-CoV-2–naive adults, this sustained induction was not evident for cTfh in SARS-CoV-2–experienced adults by comparison. However, since Tfh provides aid to B cells, these findings suggest whether B cell responses also differed by an individual's COVID-19 history (Samanovic et al., 2021).
Effect of the vaccine on SARS-CoV-2–specific ASCs in naive and experienced individuals
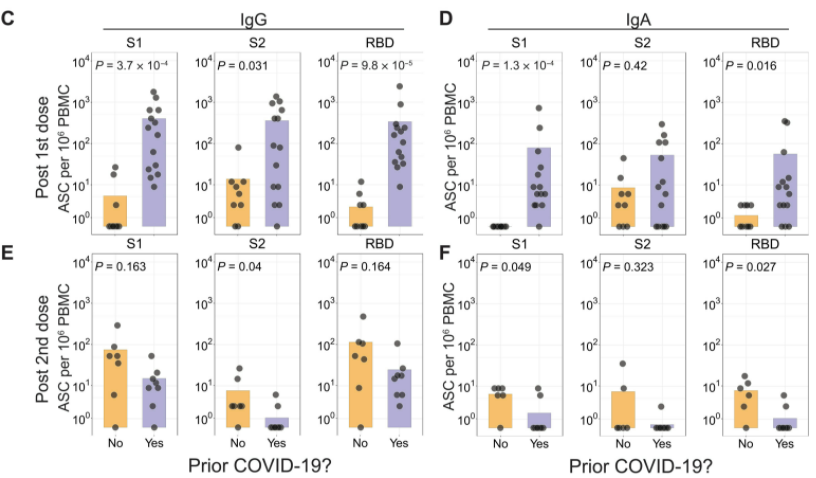
Figure 9: ASC populations measured by ELISpots in SARS-CoV-2 experienced and naive cohorts.
To test the influence of prior infection of SARS-CoV-2 on antigen-specific B cell responses, Samanovic et al. (2021) used ELISpot analyses of ASCs. These ELISpots were performed one week after each vaccine dose for both the experienced and naive cohorts. After the first dose of the vaccine, there was an increase in ASCs for SARS-CoV-2 experienced individuals, only a few SARS-CoV-2 specific ASCs were found in SARS-CoV-2 naive individuals, as can be seen in Figure 9 (Samanovic et al., 2021). Experienced cohorts showed an increase in IgG-secreting ASC that are specific to the receptor binding domain (CBD), S1 domain, and S2 domain of the spike protein (Samanovic et al., 2021). This was expected, as SARS-CoV-2-reactive B cells have been found to persist after an infection of COVID-19 (Dan et al., 2021). Additionally, IgA-secreting ASCs were identified in SARS-CoV-2 experienced adults (Samanovic et al., 2021).
The second dose induced a strong antigen-specific ASC response in both groups. However, in the second dose, there was a stronger response in the naive cohort, while the experienced showed a similar or weaker ASC response, as seen in Figure 9 (Samanovic et al., 2021). There is also a similar trend found with isotype switching, vaccine dose, and prior infection of SARS-CoV-2. Naive individuals had IgM, IgA, and IgG secreting ASCs after the second vaccination, but not the first. Experienced individuals, on the other hand, had this ASCs response after the first vaccination, but not the second. Further studies will be needed to determine what mechanism is responsible for this difference, as the results may simply reflect the low numbers of ASCs detected in the naive cohort after the first dose (Samanovic et al., 2021).
Antigen-specific B cells are induced by vaccination
To study the B cell responses after immunization, fluorescent recombinant RBD protein was used to identify RBD-reactive B cells. It was found that there was a two-fold higher baseline of RBD+ B cells in experienced individuals compared to naive ones. Additionally, experienced adults had more class-switched B cells, as they secreted less IgG than the naive adults. After the first dose, there were increased frequencies of RBD+ B cells in both groups, however, this was much higher in experienced individuals, as can be seen in Figure 10, A and B (Samanovic et al., 2021). Additionally, we see the same trend as before, where the naive cohort had a much stronger response after the second dose, while the experienced cohort had a very strong response after the first dose, but the second dose had a weakening effect. Figure 10, C and D, shows how there was an increase in isotype switching that followed the same pattern once again, evidenced by the secretion of IgD from IgG (Samanovic et al., 2021).
Figure 10 E and F show that there was an increase in CD71+ RBD+ B cells that also followed the same pattern as the RBD+ and isotype switched B cells. CD71+ was found to be induced after influenza vaccination, and is an indicator that plasmablast differentiation has occurred. Other B cell subsets such as a reduction of CD24 and CD21 were also found, which support plasmablast differentiation (Samanovic et al., 2021).
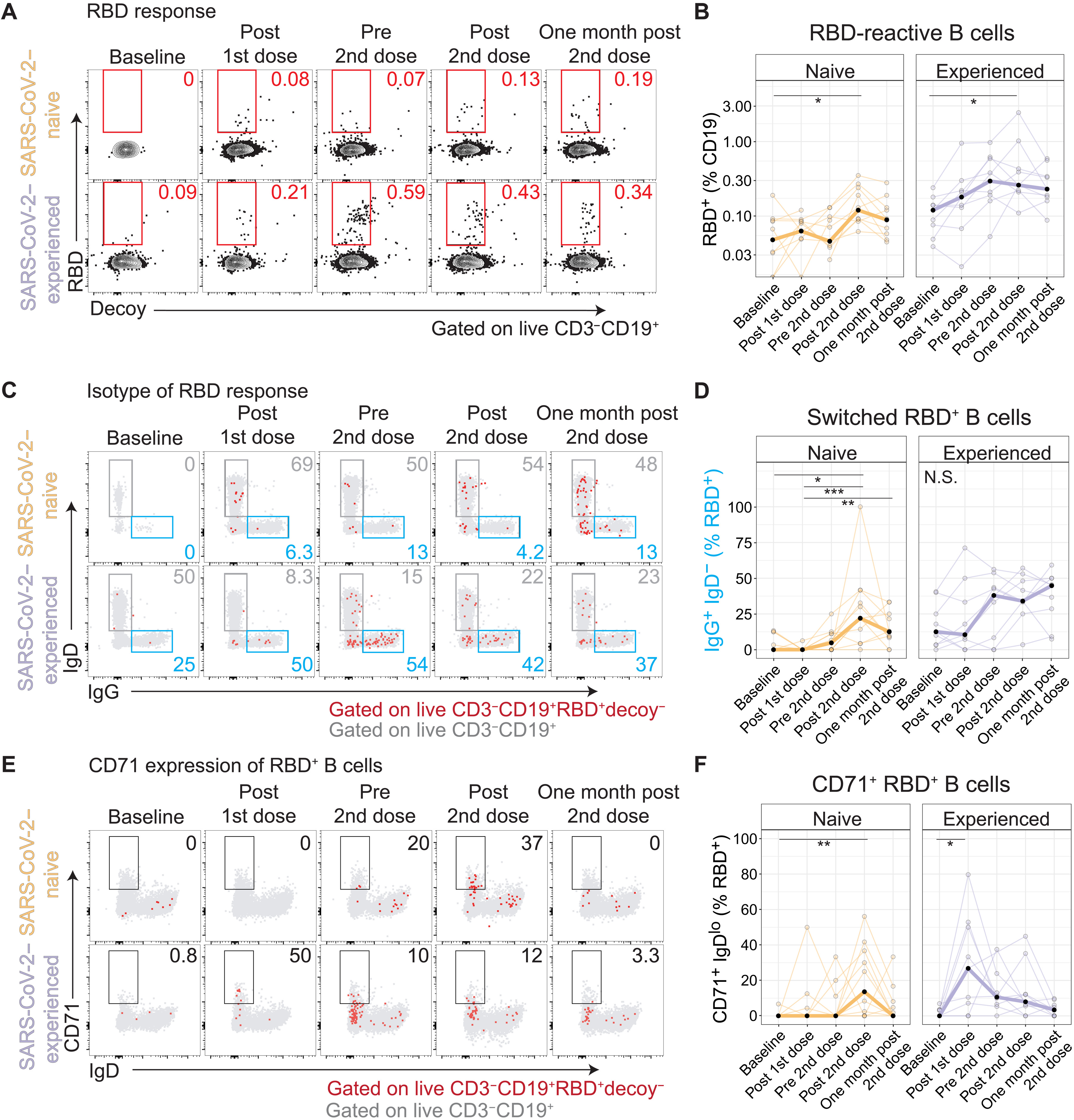
Figure 10: B cell subpopulations found in circulation of COVID-19 experienced and naive cohorts after one and two m-RNA vaccine doses.
Humoral responses differ by history of COVID-19
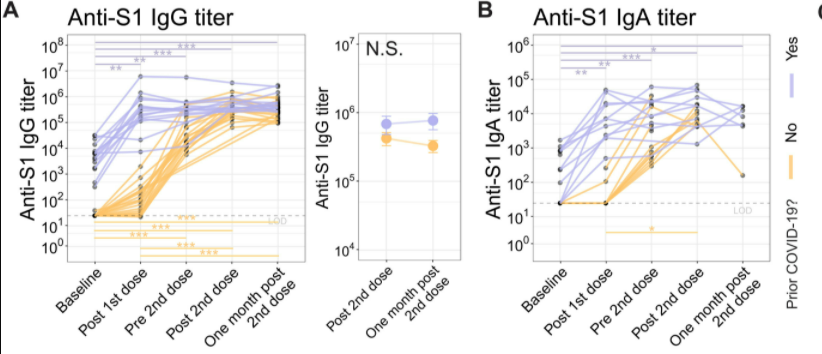
Figure 11: Anti-S1 IgG and IgA antibody response in SARS-CoV-2 experienced and naive cohorts before and after the first and second mRNA dose.
Humoral responses were investigated as they are related to ASC induction, which has been shown to differ between cohorts. To test the difference in humoral response, the S1 subunit of the spike protein was used. At baseline, anti-S1 IgG antibodies were detectable in individuals who had prior history of COVID-19, however there were no anti-S1 antibodies found in the naive cohort. After the first dose, there was a strong IgG and IgA in experienced individuals compared to the naive adults, which can be seen in Figure 11 A and B. For example, experienced individuals had a median fold change of 92 in IgG, whereas the SARS-CoV-2 naive individuals had an increase of only 2.7. Following the established pattern the second dose lead to a median fold change of 1.3 of IgG in experienced adults, but the naive individuals experienced a fold change of 11, which can also be seen in Figure 11 A and B (Samanovic et al., 2021).
Similarly, neutralizing antibodies were not detectable in SARS-CoV-2 naive adults, while they were present in low levels in experienced adults, as pictured in Figure 12 D. After the first immunization, experienced adults had a large increase in neutralizing antibodies with a median of 4084. Naive adults only had a small increase to a median of 10. However, upon second vaccination, there was a great increase of neutralizing antibodies in the naive cohort, but no significant change in the experienced cohort. Neutralizing antibodies remained at higher levels in the experienced individuals even one month after the second dose (Samanovic et al., 2021).
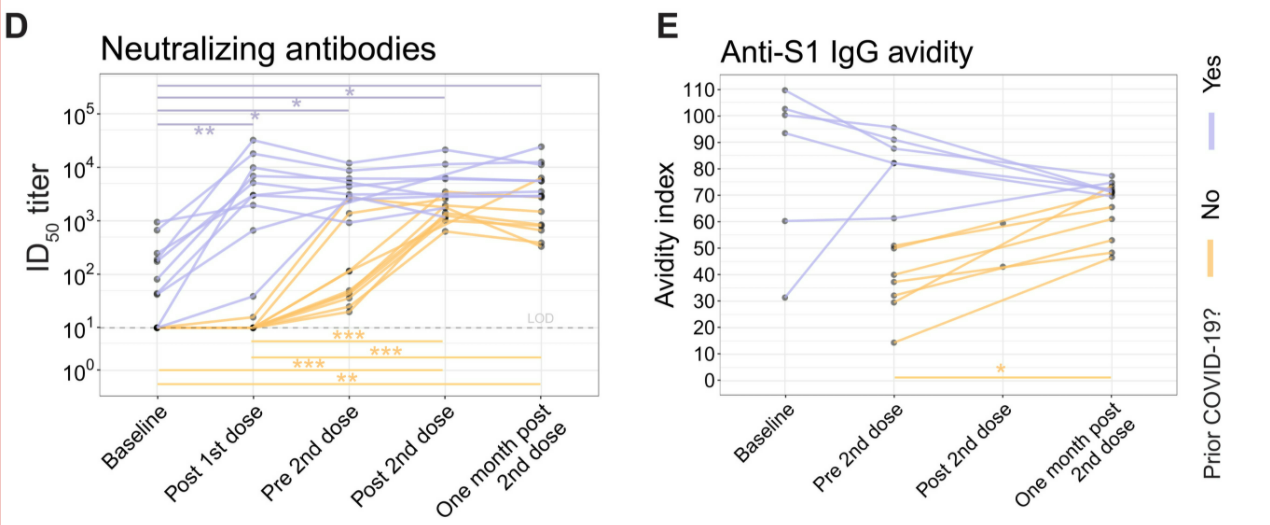
Figure 12: Neutralizing antibody titers and antibody avidity in SARS-CoV-2 experienced and naive cohorts before and after the first and second mRNA dose.
Affinity maturation occurs in the germinal center via a process called somatic hypermutation (Mesin et al., 2016). When B cells undergo somatic hypermutation, their DNA that codes for antibodies undergoes mutations which may increase their affinity for the antigen (Mesin et al., 2016). Affinity and avidity are further outlined in the discussion section. This was assessed using antibody avidity via ELISAs for anti-S1 IgG antibodies, the results of which are shown in Figure 12 E (Samanovic et al., 2021). Avidity increased steadily over time in naive adults after the first and second doses. This is expected, as it is consistent with other findings that showed that germinal center reactions last at least 5 weeks after RNA vaccination, leading to increased affinity via somatic hypermutation (Turner et al., 2021b). However, experienced adults showed a decrease in avidity over time (Samanovic et al., 2021). This may be due to an introduction of new humoral responses that have not had the time to undergo affinity maturation replacing mature high-avidity antibody secreting cells that already existed due to prior infection of COVID-19 (Samanovic et al., 2021).
Discussion
Humoral Response VS. Cell-mediated Response
Reading Tips
There are two main mechanisms of immunity within the adaptive immune system: humoral and cell-mediated immunity. Despite both immunities relying on lymphoid cells to function, they also have some important differences.
Humoral immunity is an antibody-mediated immunity that is activated when foreign antigens from bacteria and viruses are detected in the body (Dornell, 2021). This mechanism is facilitated by helper T cells and B cells that can differentiate into antibody-secreting plasma B cells (Healio, 2022). The humoral immune system mainly deals with the free-circulating pathogenetic antigens (Dornell, 2021). These Y-shaped antibodies produced by the B cells contain antigen-binding sites that specifically bind to their target antigens, neutralizing them, or causing lysis (molecular destruction) or phagocytosis (Dornell, 2021).
On the other, cell-mediated immunity occurs inside infected cells and is primarily driven by mature T cells, macrophages, and cytokines production in response to an antigen. Especially, T cellmediated immunity relies on antigen-presenting cells that contain membrane-bound MHC class I proteins that recognize target antigens of foreign pathogenic invaders (Dornell, 2021). This whole process initiated by helper T cells first release cytokines to activate T cells binding to the infected cells’ MHC-antigen complex. This allows the T cells to differentiate into a cytotoxic T cell which renders the infected cell then undergoes lysis (Healio, 2022). Therefore, the binding specificity between MHC proteins and foreign antigens is essential for naïve T cells' maturation and differentiation into a helper or cytotoxic killer T cells (Dornell, 2021).
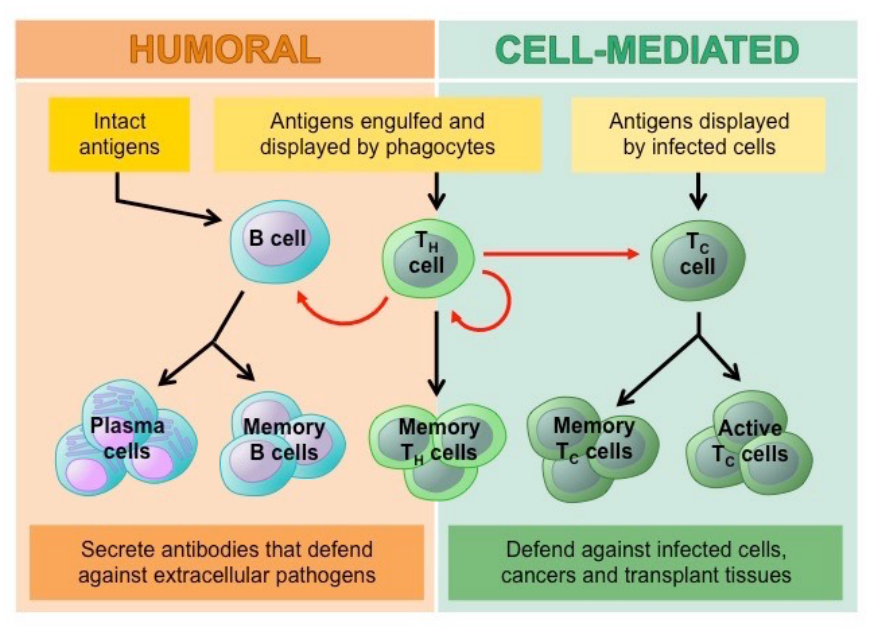
Figure 13: Humoral vs Cell mediated Immunity (Aryal, 2022).
The experimental results from the research of Samanovic et al. (2022) demonstrated similar cellular responses but different humoral responses between SARS-CoV-2 naive and SARS- CoV-2- experienced individuals.
Similar Cellular Response | Different Humoral Response |
---|---|
Induction of cytotoxic CD8+ T cells | Differential induction in antigen-specific ASCs in circulation |
Increased RBD-reactive B cell frequency in circulation | Differential increase in anti-S1 IgG antibody titers |
Table 1:Compare and Contrast of Cellular and Humoral Immune Response for Both Cohorts
Similar Cellular Response:
Both cohorts had robust induction of cytotoxic CD8+ T cells (with co-expression of Ki67 and CD38) response at a similar level following mRNA vaccination. The T cell responses were consistent with prior studies that identified antigen-specific cytotoxic T cell responses to mRNA vaccination. Additionally, there was no significant difference found in CD8+ T cell response time between the two cohorts. As for memory B cells, although RBD-specific B cells in SARS-CoV-2-experienced adults were 2-fold higher at baseline compared to SARS-CoV-2-naive adults, both cohorts RBD-reactive B cells increased in frequency in circulation following immunization.
Difference Humoral Response:
More differences were found in antigen-specific humoral cell responses. For instance, the unexpected number of antigen-specific ASCs in circulation in both groups after the second dose of vaccination. The researchers expected to find a stronger ASCs response in individuals who recovered from COVID-19 as they have more persistent SARS-CoV-2-reactive B cells. Indeed, SARS-CoV-2-experienced adults had more antigen-specific ASCs in circulation one week after the first dose compared to SARS-CoV-2-naive adults. However, the second vaccination-induced weaker ASC responses in SARS-COV- 2-experienced adults all three SARS-CoV-2 antibodies (IgM-, IgA-, and IgG-secreting ASCs) tested. Taking together, the increase in frequency for all three antibodysecreting ASCs were observed after the second vaccination but not after the first vaccination among SARS-CoV-2-naive adults, whereas similar increases were found among inexperienced adults after the first, but not after the second, vaccination. This suggested a pattern of increased induction of antigen-specific ASC responses with repeated vaccination in SARS-CoV-2-naive adults, yet the same ASCs induction might be weaker in SARS-CoV-2-experienced adults.
To further investigate whether humoral responses were affected by prior history of COVID-19, researchers assessed IgG antibody responses to the S1 subunit of the spike protein. As the result, they found a 100- to 1000-fold increase in anti-spike protein IgG antibody titers in SARS-CoVexperienced individuals upon the first dose but a limited increase after the second dose. In contrast, the IgG antibody titers increased steadily over time in SARS-CoV-2-naive adults. Thus, this experiment illustrated a rapid and robust humoral response was induced after the first vaccination in both cohorts and the further increase continued after the second dose in naive adults but minimal increase in experience adults (Samanovic et al., 2021)
Quantitative and Qualitative Differences
Reading Tips
Antibody affinity and avidity are two terms used in immunology to describe the binding strength between an antibody and antigen (Technology Networks, 2020). Since both terms describe the binding strength involved, affinity and avidity are often confused and misunderstood. Common antigens include viruses, fungi, bacteria and toxin molecules, which are called external or foreign antigens as they originate outside of the body (Technology Networks, 2020). Once detected by our body’s immune system, the immune response will be triggered by these antigens to protect ourselves from antigen-induced damages (Technology Networks, 2020). This is where antibodies come to play, as they are molecules produced by the immune system to fight antigens (Technology Networks, 2020). Antibodies function by binding to the antigens that triggered the immune response and destroy or neutralizing them (Technology Networks, 2020). Therefore, we have the terms affinity and avidity come in.
Affinity is defined as the strength of a single bond or interaction between a receptor and its respective ligand, in this case, the antibody-antigen couplet (Fluidic Analytics, 2021). Especially, it is the strength of the interaction between the antigen’s epitope and the antibody’s paratope at a singular binding site (Technology Networks, 2020). A number of factors affect this antibody-antigen binding affinity including Hydrogen bonds, ionic bonds, Van der Waals interactions and electrostatic forces (Fluidic Analytics, 2021).
On the other hand, avidity is a term that described the overall or accumulated strength of proteinprotein complex interaction (Fluidic Analytics, 2021). Therefore, antibody avidity is the measure of the total binding strength of an antibody at every binding site because antibodies and antigens are multivalent with more than one binding site (Technology Networks, 2020). It is determined by three parameters:
- Ordered List ItemThe binding affinity of the complex: The strength of the relationship at a singular binding site between the antibody-antigen couplet.
- The valency of the proteins: The total number of binding sites involved in the antibody-antigen couplet.
- The structural arrangement of the proteins in the complex: The structure of the antigen and antibody involved.
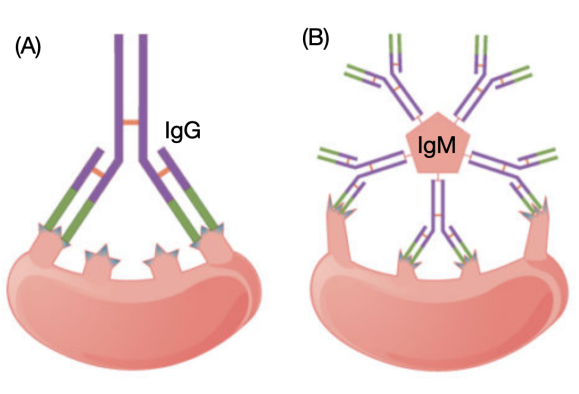
Figure 14: **Affinity VS Avidity.** (A) Affinity refers to the strength of single interaction between antigen and antibody: each IgG binding site has a high affinity to its target antigen epitope. (B) Avidity refers to the strength of all interactions combined: although IgM has a low-affinity binding site, the sum of ten binding sites gives it total high binding avidity. Image adapted from (Ivy Tech & Lumen Learning, n.d.).
In the study of Samanovic et al. (2022), qualitative and quantitative differences in immune responses, especially humoral responses were observed in naive and previously infected SARSCoV-2 individuals.
Quantitative | Qualitative |
---|---|
Neutralizing antibodies titers increased 400-fold higher in experienced individuals | Avidity for spike protein decreased in experienced individuals |
Table 1: Quantitative and Qualitative Difference in Immune Response Between Two Cohorts
Quantitative Difference
Following the first immunization, the number of live-virus neutralizing antibodies titers increased in SARS-CoV-2-experienced adults was 400-fold higher (4084 VS. 10) compared to SARS-CoV-2-naive adults. Similar to the anti-spike protein IgG antibody, subsequent neutralizing antibody titers were maintained at a similar level by second vaccination in SARS-CoV- 2-experienced adults, whereas the neutralizing titers in SARS-CoV-2-naive adults continued to increase. Nonetheless, owing to the huge quantitative difference post 1st dose, neutralizing antibody responses in the SARS-CoV-2-experienced group still remained greater magnitude compared to the naive group after the second vaccination and even one month after.
Qualitative Difference
Additionally, the avidity to viral spike protein could change qualitatively despite the plateau in antibody quantity. In SARS-CoV-2-naive adults, antibody avidity to spike protein continued to increase steadily the whole immunization process and even one-month post 2nd dose when antibody titers had plateaued. This prolonged period of increasing avidity is consistent with other reports of human germinal center-related affinity maturation lasting at least 5 weeks post-RNA vaccination. In contrast, despite SARS-CoV-2-experienced adults having relatively high baseline antibodies avidity, the antibody affinity decreased over time and with second vaccination. One speculation would be the de novo B cell responses following vaccination among experienced adults induced new, low avidity humoral responses that had not undergone germinal center maturation (Samanovic et al., 2021).
Implications: This paper gave us a better understanding of the qualitative and quantitative changes in antibodies over time in both SARS-CoV-2-naive and experienced individuals. The results will have major implications for the future investigation addressing the question of the need for booster vaccinations. For example, whether booster doses will lead to higher titers of antibodies or the antibodies will plateau at a certain threshold?
The Question:
Question: Why humoral responses were robust after the first vaccination but more muted after the second vaccination in SARS-CoV-2-experienced adults?
The Speculations (Samanovic et al., 2021):
- Ordered List ItemThe early plateau in humoral responses in Covid experienced individuals suggested an altered B cell differentiation away from antigen-specific plasma cells. For example, the reduced antigen-specific ASCs responses may alter the trafficking of ASCs following repeat vaccination. This, in turn, shifts the peak ASC response earlier to the experimental assessment time.
- The high titers of anti-S1 IgG responses may restrict further antigen availability to stimulate non-memory B cell clones following subsequent vaccine doses.
- Pre-existed differences between two cohorts could arise prior to humoral response activation, such as antigen-presenting cell priming and duration innate immune responses in the setting of novel COVID-19.
Conclusion
Application of Research
Personalized Vaccines
The research by Samanovic et al. (2021) has a wide ranges of applications that can help shape the future research and development of vaccines. Samanovic et al. (2021) strongly emphasizes in their paper that personalized vaccines against COVID-19 may help in prolonging immunity. Currently, vaccines are deployed under the “same dose fits all” model, which some argue retains the post-WWII mentality of vaccine administration (Poland et al., 2018). Prior to the paper by Samanovic et al. (2021), there has been a lack of research pertaining to immune response following vaccination; this has contributed to immuno-immaturity and the poor immunogenicity of vaccines for the elderly populations (Poland et al., 2018). With such research by Samanovic et al. (2021), researchers now understand the exact immunological response that occurs in the human body for both SARS-CoV-2 experienced and SARS-CoV-2 naive individuals. Thereby, the research by Samanovic et al. (2021) provides insight on how personalized vaccines can aid in prolonging immunity for SARS-CoV-2 experienced and SARS-CoV-2 naive individual.
Booster Shots
The research by Samanovic et al. (2021) provides insight into the deployment of booster shots for COVID-19. It is widely understood that waning immunity often occurs after administration of BNT162b2 for both SARS-CoV-2 experienced and SARS-CoV-2 naive individuals (Levin et al., 2021; Samanovic et al., 2021). The results from Samanovic et al. (2021) provides an avenue to future vaccine development and consideration for the deployment of booster shots. Perhaps, those who have experienced COVID-19 may need a different dosage than SARS-CoV-2 naive individuals, according to Poland et al. (2018). Future studies, however, must be deployed to investigate the efficiency of booster shots for SARS-CoV-2 experienced and SARS-CoV-2 naive individuals. Other future studies may also need to be deployed to understand if booster shots need to be administrated annually akin to flu shots for both SARS-CoV-2 experienced and SARS-CoV-2 naive individuals.
Experimental Flaws and Critiques
Small Sample Size
Before real-world applications can be applied, it is important to address experimental flaws that are present in the research. To begin, Samanovic et al. (2021) utilizes an extremely small group of participants (n=36) for their research. The composition of these participants include15 adults classified as SARS-CoV-2 experienced, and 21 individuals classified as SARS-CoV-2 naive (Samanovic et al., 2021). It is without doubt that this sample size is far too small to conclusively gain a proper insight into the immunological response that occurs for SARS-CoV-2 experienced and SARS-CoV-2 naive individuals. It is important to note that each participant in this study was an adult, and will not accurately depict the result of an individual with a weakened immune system, such as the elderly (Montecino-Rodriguez et al., 2013). Any disparities in such a small group of individuals can greatly skew the data.
SARS-CoV-2 Variant
In addition, Samanovic et al. (2021) failed to disclose the particular variant of SARS-CoV-2 the experienced individuals were infected with. It is known that SARS-CoV-2 is constantly evolving, causing mutations within the receptor binding domain (Becker et al., 2021). In a study by Becker et al. (2021), results have shown that the immune response towards the Omicron variant of SARS-CoV-2 greatly differ in comparison to the firstly-emerged SARS-CoV-2 virus.
Peripheral Immune Response Measurements
Finally, Samanovic et al. (2021) measured the immune peripheral response after 1 week of the first and second dose of BNT162b2. Much more accurate results could be obtained, should Samanovic et al. (2021) collect peripheral response data on a daily basis.
Future Directions
The results by Samanovic et al. (2021) can be used to discuss future directions pertaining to the research and development of vaccines. These future directions include:
- Future studies that analyze the correlation between the spike protein-specific T cells that are induced after BNT162b2 administration, and protection (Samanovic et al., 2021). In essence, we must understand if T cells are an appropriate measurement of protection (Samanovic et al., 2021).
- Future studies that analyze whether booster shots are capable of increasing the antibody titer, or only result in an increase to threshold (Samanovic et al., 2021). An increase to threshold was observed in the results for SARS-CoV-2 experienced individuals (Samanovic et al., 2021).
- Future studies that analyze the impact of our pre-existing T cells, B cells and post-infection immunity due to seasonal coronaviruses (Samanovic et al., 2021). By performing this experiment, it provides insight as to how researchers can improve the ability to develop effective COVID-19 vaccines.
- It is clear the differential responses pertaining to avidity and somatic hypermutation occurred in the research by Samanovic et al. (2021). Future studies that investigate the mechanism of the secondary lymphoid organs may help us understand more upon the results that Samanovic et al. (2021) achieved.
All in all, the research by Samanovic et al. (2021) provide insight into the immune response observed in SARS-CoV-2 experienced and SARS-CoV-2 naive individuals that can be used as an avenue to facilitate and enhance future vaccine development.
References
Aryal, S. (2022, January 25). Humoral vs Cell mediated Immunity- Definition, 20 Differences. Microbe Notes. https://microbenotes.com/differences-between-humoral-immunity-and-cell-mediated-immunity
Bentebibel, S. E., Lopez, S., Obermoser, G., Schmitt, N., Mueller, C., Harrod, C., … Ueno, H. (2013). Induction of ICOS+CXCR3+CXCR5+ TH cells correlates with antibody responses to influenza vaccination. Science Translational Medicine, 5(176). https://doi.org/10.1126/SCITRANSLMED.3005191
Becker, M., Dulovic, A., Junker, D., Ruetalo, N., Kaiser, P. D., Pinilla, Y. T., Heinzel, C., Haering, J., Traenkle, B., Wagner, T. R., Layer, M., Mehrlaender, M., Mirakaj, V., Held, J., Planatscher, H., Schenke-Layland, K., Krause, G., Strengert, M., Bakchoul, T., Althaus, K., … Schneiderhan-Marra, N. (2021). Immune response to SARS-CoV-2 variants of concern in vaccinated individuals. Nature communications, 12(1), 3109. https://doi.org/10.1038/s41467-021-23473-6
BioRender. (2022). Create Professional Science Figures in Minutes. BioRender. Retrieved March 1, 2022, from https://biorender.com/
CDC. (2022). Understanding mRNA COVID-19 Vaccines. Centers for Disease Control and Prevention. Retrieved March 1, 2022 from https://www.cdc.gov/coronavirus/2019-ncov/vaccines/different-vaccines/mrna.html
Dan, J. M., Mateus, J., Kato, Y., Hastie, K. M., Yu, E. D., Faliti, C. E., . . . Crotty, S. (2021). Immunological memory to SARS-COV-2 assessed for up to 8 months after infection. Science, 371(6529). doi:10.1126/science.abf4063
Dornell, J., PhD. (2021, June 15). Humoral vs Cell-Mediated Immunity. From Technology Networks. https://www.technologynetworks.com/immunology/articles/humoral-vs-cell-mediated-immunity-344829
ELISpot Assay. (2022). Retrieved 3 March 2022, from https://www.rndsystems.com/products/elispot-assay-principle
Flaxman, A., & Ewer, K. (2018). Methods for Measuring T-Cell Memory to Vaccination: From Mouse to Man. Vaccines, 6(3), 43. doi: 10.3390/vaccines6030043
Fluidic Analytics. (2021, August 24). Affinity vs Avidity — What’s the difference? https://www.fluidic.com/resources/affinity-vs-avidity-whats-the-difference/
Healio. (2022). Adaptive Immunity – Humoral and Cellular Immunity. Learn ImmunoOncology. Retrieved February 28, 2022, from https://www.healio.com/hematology-oncology/learn-immuno-oncology/the-immune-system/adaptive-immunity-humoral-and-cellular-immunity
Herati, R. S., Reuter, M. A., Dolfi, D. V., Mansfield, K. D., Aung, H., Badwan, O. Z., … Wherry, E. J. (2014). Circulating CXCR5+PD-1+ response predicts influenza vaccine antibody responses in young adults but not elderly adults. Journal of Immunology (Baltimore, Md. : 1950), 193(7), 3528–3537. https://doi.org/10.4049/JIMMUNOL.1302503
How does flow cytometry work? Nanocellect. (2021, June 17). Retrieved March 3, 2022, from https://nanocellect.com/blog/how-does-flow-cytometry-work/#:~:text=Flow%20Cytometry%20is%20a%20technique,into%20the%20flow%20cytometer%20instrument
Ivy Tech & Lumen Learning. (n.d.). Antibodies | Biology I. Lumenlearning. Retrieved March 1, 2022, from https://courses.lumenlearning.com/ivytech-bio1-1/chapter/antibodies/ Kovarik, J., Kämpf, A., Gasser, F., Herdina, A., Breuer, M., & Kaltenecker, C. et al. (2021). Identification of Immune Activation Markers in the Early Onset of COVID-19 Infection. Frontiers In Cellular And Infection Microbiology, 11. doi: 10.3389/fcimb.2021.651484
Mesin, L., Ersching, J., & Victora, G. (2016). Germinal center B cell dynamics. Immunity, 45(3), 471-482. doi:10.1016/j.immuni.2016.09.001
Montecino-Rodriguez, E., Berent-Maoz, B., & Dorshkind, K. (2013). Causes, consequences, and reversal of immune system aging. The Journal of clinical investigation, 123(3), 958–965. https://doi.org/10.1172/JCI64096
Pfizer. (n.d.). Coronavirus COVID-19 Vaccine Updates. Pfizier. Retrieved March 1, 2022 from https://www.pfizer.com/science/coronavirus/vaccine/rapid-progress
Poland, G. A., Ovsyannikova, I. G., & Kennedy, R. B. (2018). Personalized vaccinology: A review. Vaccine, 36(36), 5350–5357. https://doi.org/10.1016/j.vaccine.2017.07.062
Reiss, S., Baxter, A., Cirelli, K., Dan, J., Morou, A., & Daigneault, A. et al. (2017). Comparative analysis of activation induced marker (AIM) assays for sensitive identification of antigen-specific CD4 T cells. PLOS ONE, 12(10), e0186998. doi: 10.1371/journal.pone.0186998
Rudnick, S. I., & Adams, G. P. (2009). Affinity and avidity in antibody-based tumor targeting. Cancer Biotherapy and Radiopharmaceuticals, 24(2), 155-161. doi:10.1089/cbr.2009.0627
Samanovic, M. I., Cornelius, A. R., Gray-Gaillard, S. L., Allen, J. R., Karmacharya, T., Wilson, J. P., Wesley Hyman, S., Tuen, M., Koralov, S. B., Mulligan, M. J., & Sedaghat Herati, R. (2021). Robust immune responses are observed after one dose of BNT162b2 mRNA vaccine dose in SARS-CoV-2 experienced individuals. Science translational medicine, 14(631). doi:19.1126/scitranslmed.abi8961
Sahin, U., Muik, A., Derhovanessian, E., Vogler, I., Kranz, L. M., Vormehr, M., … Türeci, Ö. (2020). COVID-19 vaccine BNT162b1 elicits human antibody and TH1 T cell responses. Nature 2020 586:7830, 586(7830), 594–599. https://doi.org/10.1038/s41586-020-2814-7
Spicer, K. B., Glick, C., Cavanaugh, A. M., & Thoroughman, D. (2022). Protective Immunity after Natural Infection with Severe Acute Respiratory Syndrome Coronavirus-2 (SARS-CoV-2) - Kentucky, USA, 2020. International journal of infectious diseases : IJID : official publication of the International Society for Infectious Diseases, 114, 21–28. https://doi.org/10.1016/j.ijid.2021.10.010
Technology Networks. (2020, April 17). Affinity vs Avidity. From Technology Networks. https://www.technologynetworks.com/immunology/articles/affinity-vs-avidity-333559 Turner, J. S., Kim, W., Kalaidina, E., Goss, C. W., Rauseo, A. M., Schmitz, A. J., Hansen, L., Haile, A., Klebert, M. K., Pusic, I., O'Halloran, J. A., Presti, R. M., & Ellebedy, A. H. (2021a). SARS-CoV-2 infection induces long-lived bone marrow plasma cells in humans. Nature, 595(7867), 421–425. https://doi.org/10.1038/s41586-021-03647-4
Turner, J. S., O’Halloran, J. A., Kalaidina, E., Kim, W., Schmitz, A. J., Zhou, J. Q., . . . Ellebedy, A. H. (2021b). SARS-COV-2 mrna vaccines induce persistent human germinal centre responses. Nature, 596(7870), 109-113. doi:10.1038/s41586-021-03738-2
WHO. (2022). WHO Coronavirus (COVID-19) Dashboard. World Health Organization. Retrieved March 1, 2022 from https://covid19.who.int/